Sustainable pathways for biomass production and utilization in carbon capture and storage—a review
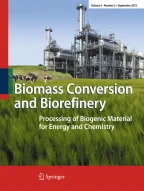
The urgency to mitigate greenhouse gas emissions has catalyzed interest in sustainable biomass production and utilization coupled with carbon capture and storage (CCS). This review explores diverse facets of biomass production, encompassing dedicated energy crops, agricultural residues, and forest residues, along with sustainable production practices and land management strategies. Technological advancements aimed at enhancing biomass yields, including precision agriculture, genetic engineering, and advanced processing technologies, are examined. Thermochemical methods (gasification, pyrolysis) and biochemical methods (anaerobic digestion, fermentation) for biomass conversion are detailed, highlighting their roles in biomass utilization. Integrated biorefineries are emphasized for maximizing biomass efficiency. The review thoroughly covers CCS, including CO2 capture and transport advancements, innovative storage solutions, and challenges in implementation. Bioenergy with carbon capture and storage (BECCS) strategies for achieving negative emissions are discussed, with insights from case studies like the BIO-CAP-UK project and initiatives in New South Wales, Australia. This review provides a comprehensive overview of sustainable biomass pathways and their critical role in CCS, offering insights into current technologies, limitations, and concluding with implications for climate change mitigation strategies.
This is a preview of subscription content, log in via an institution to check access.
Access this article
Subscribe and save
Springer+ Basic
€32.70 /Month
- Get 10 units per month
- Download Article/Chapter or eBook
- 1 Unit = 1 Article or 1 Chapter
- Cancel anytime
Buy Now
Price includes VAT (France)
Instant access to the full article PDF.
Rent this article via DeepDyve
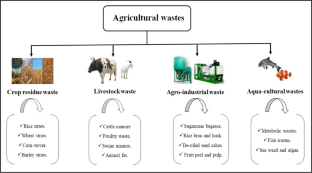
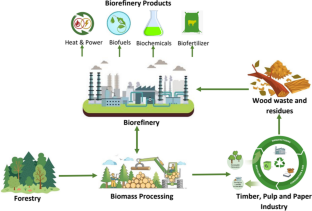
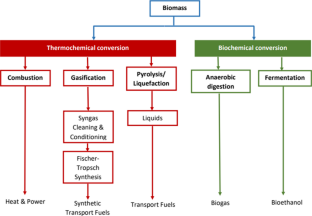
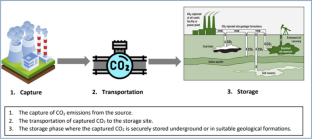
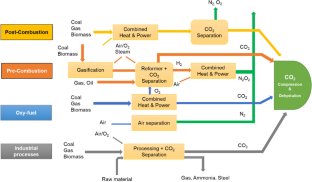
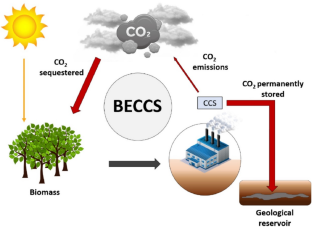
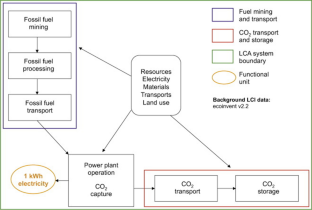
Similar content being viewed by others
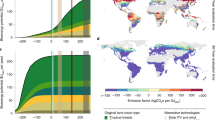
The climate change mitigation potential of bioenergy with carbon capture and storage
Article 24 August 2020
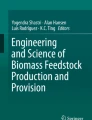
Biomass Feedstock Production and Provision: Overview, Current Status, and Challenges
Chapter © 2014
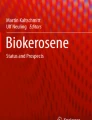
Potentials of Biomass and Renewable Energy: The Question of Sustainable Availability
Chapter © 2018
Explore related subjects
References
- Loucks DP (2021) Impacts of climate change on economies, ecosystems, energy, environments, and human equity: a systems perspective. In: The impacts of climate change. Elsevier, pp 19–50 ChapterGoogle Scholar
- Serafin J, Dziejarski B, Solis C, de la Piscina PR, Homs N (2024) Medium-pressure hydrogen storage on activated carbon derived from biomass conversion. Fuel 363:130975 ArticleGoogle Scholar
- Kumba H, Olanrewaju OA (2024) ‘Towards sustainable development: analyzing the viability and integration of renewable energy solutions in South Africa’—a review. Energies 17(6):1418 ArticleGoogle Scholar
- Sánchez J, Curt MD, Robert N, Fernández J (2019) Biomass resources. In: The role of bioenergy in the bioeconomy. Elsevier, pp 25–111 ChapterGoogle Scholar
- Subbarao PMV, D’Silva TC, Adlak K, Kumar S, Chandra R, Vijay VK (2023) Anaerobic digestion as a sustainable technology for efficiently utilizing biomass in the context of carbon neutrality and circular economy. Environ Res:116286
- Gür TM (2022) Carbon dioxide emissions, capture, storage and utilization: review of materials, processes and technologies. Prog Energy Combust Sci 89:100965 ArticleGoogle Scholar
- Sri Shalini S, Palanivelu K, Ramachandran A, Raghavan V (2021) Biochar from biomass waste as a renewable carbon material for climate change mitigation in reducing greenhouse gas emissions—a review. Biomass Convers Biorefinery 11(5):2247–2267 ArticleGoogle Scholar
- García-Freites S, Gough C, Röder M (2021) The greenhouse gas removal potential of bioenergy with carbon capture and storage (BECCS) to support the UK’s net-zero emission target. Biomass Bioenergy 151:106164 ArticleGoogle Scholar
- Yang F, Meerman JC, Faaij APC (2021) Carbon capture and biomass in industry: a techno-economic analysis and comparison of negative emission options. Renew Sust Energ Rev 144:111028 ArticleGoogle Scholar
- Abdullah B et al (2019) Fourth generation biofuel: a review on risks and mitigation strategies. Renew Sust Energ Rev 107:37–50 ArticleGoogle Scholar
- Cao L et al (2020) Biorenewable hydrogen production through biomass gasification: a review and future prospects. Environ Res 186:109547 ArticleGoogle Scholar
- Mendiara T et al (2018) Negative CO2 emissions through the use of biofuels in chemical looping technology: a review. Appl Energy 232:657–684 ArticleGoogle Scholar
- Antar M, Lyu D, Nazari M, Shah A, Zhou X, Smith DL (2021) Biomass for a sustainable bioeconomy: an overview of world biomass production and utilization. Renew Sust Energ Rev 139:110691 ArticleGoogle Scholar
- Tanzer SE, Blok K, Ramirez A (2021) Decarbonising industry via BECCS: promising sectors, challenges, and techno-economic limits of negative emissions. Curr Sustain Renew Energy Rep:1–10
- Shahbaz M et al (2021) A comprehensive review of biomass based thermochemical conversion technologies integrated with CO2 capture and utilisation within BECCS networks. Resour Conserv Recycl 173:105734 ArticleGoogle Scholar
- Hafezi R, Alipour M (2021) Renewable energy sources: traditional and modern-age technologies. In: Affordable and clean energy. Springer, pp 1085–1099 ChapterGoogle Scholar
- Rial RC (2024) Biofuels versus climate change: exploring potentials and challenges in the energy transition. Renew Sust Energ Rev 196:114369 ArticleGoogle Scholar
- Pathak G, Dudhagi SS (2021) Bioenergy crops as an alternate energy resource. Bioprospect Plant Biodiversity Ind Mol:357–376
- Vera I, Hoefnagels R, Junginger M, van der Hilst F (2021) Supply potential of lignocellulosic energy crops grown on marginal land and greenhouse gas footprint of advanced biofuels—a spatially explicit assessment under the sustainability criteria of the Renewable Energy Directive Recast. GCB Bioenergy 13(9):1425–1447 ArticleGoogle Scholar
- “Bioenergy crops in England and the UK: 2008-2023,” GOV.UK. Accessed: Jun. 25, 2024. Available: https://www.gov.uk/government/statistics/bioenergy-crops-in-england-and-the-uk-2008-2023/bioenergy-crops-in-england-and-the-uk-2008-2023
- Agu OS, Tabil LG, Mupondwa E, Emadi B, Dumonceaux T (2022) Impact of biochar addition in microwave torrefaction of camelina straw and switchgrass for biofuel production. Fuels 3(4):588–606 ArticleGoogle Scholar
- Ruatpuia JVL et al (2023) Green biodiesel production from Jatropha curcas oil using a carbon-based solid acid catalyst: a process optimization study. Renew Energy 206:597–608 ArticleGoogle Scholar
- Makepa DC, Fumhirwa DV, Tambula S, Chihobo CH (2024) Performance analysis, techno-economic and life cycle assessment of Jatropha curcas L.(Euphorbiaceae) seedcake gasification and Fischer-Tropsch integrated process for bio-methanol production. Biofuels 15(1):57–66 ArticleGoogle Scholar
- Vasconcelos MH et al (2020) Techno-economic assessment of bioenergy and biofuel production in integrated sugarcane biorefinery: identification of technological bottlenecks and economic feasibility of dilute acid pretreatment. Energy 199:117422 ArticleGoogle Scholar
- Pandey A, Gupta A, Sunny A, Kumar S, Srivastava S (2020) Multi-objective optimization of media components for improved algae biomass, fatty acid and starch biosynthesis from Scenedesmus sp. ASK22 using desirability function approach. Renew Energy 150:476–486 ArticleGoogle Scholar
- Wang C, Kong Y, Hu R, Zhou G (2021) Miscanthus: a fast-growing crop for environmental remediation and biofuel production. GCB Bioenergy 13(1):58–69 ArticleGoogle Scholar
- Neupane D, Lohaus RH, Solomon JKQ, Cushman JC (2022) Realizing the potential of camelina sativa as a bioenergy crop for a changing global climate. Plants 11(6):772 ArticleGoogle Scholar
- An Y et al (2021) Opportunities and barriers for biofuel and bioenergy production from poplar. GCB Bioenergy 13(6):905–913 ArticleGoogle Scholar
- Muhammad S et al (2022) Insights into agricultural-waste-based nano-activated carbon fabrication and modifications for wastewater treatment application. Agriculture 12(10):1737 ArticleGoogle Scholar
- Patra D, Patra BR, Pattnaik F, Hans N, Kushwaha A (2022) Recent evolution in green technologies for effective valorization of food and agricultural wastes. In: Emerging Trends to Approaching Zero Waste. Elsevier, pp 103–132 ChapterGoogle Scholar
- Ma Y, Shen Y, Liu Y (2020) State of the art of straw treatment technology: challenges and solutions forward. Bioresour Technol 313:123656 ArticleGoogle Scholar
- Ndayisenga F et al (2021) Microbial electrohydrogenesis cell and dark fermentation integrated system enhances biohydrogen production from lignocellulosic agricultural wastes: substrate pretreatment towards optimization. Renew Sust Energ Rev 145:111078 ArticleGoogle Scholar
- Tripathi N, Hills CD, Singh RS, Atkinson CJ (2019) Biomass waste utilisation in low-carbon products: harnessing a major potential resource. NPJ Clim Atmos Sci 2(1):35 ArticleGoogle Scholar
- Singh P, Singh TP, Sharma RK, Negi YK, Pal R (2021) Potential of pine needle biomass as an alternative fuel to mitigate forest fire in Uttarakhand Himalayas-a review. J Agric Eng 58(2):192–203 Google Scholar
- Stafford W et al (2020) Forestry biorefineries. Renew Energy 154:461–475 ArticleGoogle Scholar
- Titus BD et al (2021) Sustainable forest biomass: a review of current residue harvesting guidelines. Energy Sustain Soc 11:1–32 Google Scholar
- Makepa DC, Chihobo CH, Musademba D (2023) Advances in sustainable biofuel production from fast pyrolysis of lignocellulosic biomass. Biofuels 14(5):529–550 ArticleGoogle Scholar
- So HW, Lafortezza R (2022) Reviewing the impacts of eco-labelling of forest products on different dimensions of sustainability in Europe. Forest Policy Econ 145:102851 ArticleGoogle Scholar
- Kumar A et al (2022) Geospatial perspectives of sustainable forest management to enhance ecosystem services and livelihood security. Adv Remote Sens For Monit:10–42
- Sikkema R, Junginger M, Van Dam J, Stegeman G, Durrant D, Faaij A (2014) Legal harvesting, sustainable sourcing and cascaded use of wood for bioenergy: their coverage through existing certification frameworks for sustainable forest management. Forests 5(9):2163–2211 ArticleGoogle Scholar
- Goushehgir Z, Feghhi J, Innes JL (2022) Challenges facing the improvement of forest management in the Hyrcanian forests of Iran. Forests 13(12):2180 ArticleGoogle Scholar
- Ranius T et al (2018) The effects of logging residue extraction for energy on ecosystem services and biodiversity: a synthesis. J Environ Manag 209:409–425 ArticleGoogle Scholar
- Calvin K et al (2021) Bioenergy for climate change mitigation: scale and sustainability. GCB Bioenergy 13(9):1346–1371 ArticleGoogle Scholar
- Ellis EA et al (2019) Reduced-impact logging practices reduce forest disturbance and carbon emissions in community managed forests on the Yucatán Peninsula, Mexico. For Ecol Manag 437:396–410 ArticleGoogle Scholar
- Collison BR, Gromack AG, Branch AE (2022) Importance of riparian zone management for freshwater fish and fish habitat protection: analysis and recommendations in Nova Scotia, Canada. Fisheries and Oceans Canada, Maritimes Region, Bedford Institute of Oceanography Google Scholar
- Kuglerová L, Muotka T, Chellaiah D, Jyväsjärvi J, Richardson JS (2024) Protecting our streams by defining measurable targets for riparian management in a forestry context. J Appl Ecol 61(2):206–214 ArticleGoogle Scholar
- H. Khan, A. A. Farooque, B. Acharya, and T. Esau, “Effect of within field soil variability on potato tuber yield,” in 2019 ASABE Annual International Meeting, American Society of Agricultural and Biological Engineers, 2019, p. 1.
- Gawande V et al (2023) Potential of precision farming technologies for eco-friendly agriculture. Int J Plant Soil Sci 35(19):101–112 ArticleGoogle Scholar
- Ahmad SF, Dar AH (2020) Precision farming for resource use efficiency. Resour Use Effic Agric:109–135
- Chao Z, Liu N, Zhang P, Ying T, Song K (2019) Estimation methods developing with remote sensing information for energy crop biomass: a comparative review. Biomass Bioenergy 122:414–425 ArticleGoogle Scholar
- Usama M (2024) Application of digital technologies & remote sensing in precision agriculture for sustainable crop production. In: Geospatial Technology to Support Communities and Policy: Pathways to Resiliency. Springer, pp 203–223 ChapterGoogle Scholar
- Rao MJ, Wang L (2021) CRISPR/Cas9 technology for improving agronomic traits and future prospective in agriculture. Planta 254:1–16 ArticleGoogle Scholar
- Pancaldi F, Trindade LM (2020) Marginal lands to grow novel bio-based crops: a plant breeding perspective. Front Plant Sci 11:496406 ArticleGoogle Scholar
- Sankaran R et al (2020) Recent advances in the pretreatment of microalgal and lignocellulosic biomass: a comprehensive review. Bioresour Technol 298:122476 ArticleGoogle Scholar
- Khan MU, Usman M, Ashraf MA, Dutta N, Luo G, Zhang S (2022) A review of recent advancements in pretreatment techniques of lignocellulosic materials for biogas production: opportunities and Limitations. Chem Eng J Adv 10:100263 ArticleGoogle Scholar
- Lee D et al (2022) Recent progress in the catalytic thermochemical conversion process of biomass for biofuels. Chem Eng J 447:137501 ArticleGoogle Scholar
- Dutta N, Usman M, Ashraf MA, Luo G, Gamal El-Din M, Zhang S (2023) Methods to convert lignocellulosic waste into biohydrogen, biogas, bioethanol, biodiesel and value-added chemicals: a review. Environ Chem Lett 21(2):803–820 ArticleGoogle Scholar
- Marangwanda GT, Madyira DM, Babarinde TO (2020) Combustion models for biomass: a review. Energy Rep 6:664–672 ArticleGoogle Scholar
- Chen W-H et al (2021) Progress in biomass torrefaction: principles, applications and challenges. Prog Energy Combust Sci 82:100887 ArticleGoogle Scholar
- Tezer Ö, Karabağ N, Öngen A, Çolpan CÖ, Ayol A (2022) Biomass gasification for sustainable energy production: a review. Int J Hydrog Energy 47(34):15419–15433 ArticleGoogle Scholar
- Babatabar MA, Saidi M (2021) Hydrogen production via integrated configuration of steam gasification process of biomass and water-gas shift reaction: process simulation and optimization. Int J Energy Res 45(13):19378–19394 ArticleGoogle Scholar
- Makepa DC, Chihobo CH, Musademba D (2023) Microwave-assisted pyrolysis of pine sawdust (Pinus patula) with subsequent bio-oil transesterification for biodiesel production. Biofuels:1–9
- Dziejarski B et al (2024) Upgrading recovered carbon black (rCB) from industrial-scale end-of-life tires (ELTs) pyrolysis to activated carbons: material characterization and CO2 capture abilities. Environ Res 247:118169 ArticleGoogle Scholar
- Yogalakshmi KN et al (2022) Lignocellulosic biomass-based pyrolysis: a comprehensive review. Chemosphere 286:131824 ArticleGoogle Scholar
- Hassan NS, Jalil AA, Hitam CNC, Vo DVN, Nabgan W (2020) Biofuels and renewable chemicals production by catalytic pyrolysis of cellulose: a review. Environ Chem Lett 18:1625–1648 ArticleGoogle Scholar
- Choi SH, Manousiouthakis VI (2020) On the carbon cycle impact of combustion of harvested plant biomass vs. fossil carbon resources. Comput Chem Eng 140:106942 ArticleGoogle Scholar
- Kunatsa T, Xia X (2022) A review on anaerobic digestion with focus on the role of biomass co-digestion, modelling and optimisation on biogas production and enhancement. Bioresour Technol 344:126311 ArticleGoogle Scholar
- Verma N, Kumar V, Bansal MC (2021) Valorization of waste biomass in fermentative production of cellulases: a review. Waste Biomass Valor 12:613–640 ArticleGoogle Scholar
- Ajayi-Banji A, Rahman S (2022) A review of process parameters influence in solid-state anaerobic digestion: focus on performance stability thresholds. Renew Sust Energ Rev 167:112756 ArticleGoogle Scholar
- Amin FR, Khalid H, El-Mashad HM, Chen C, Liu G, Zhang R (2021) Functions of bacteria and archaea participating in the bioconversion of organic waste for methane production. Sci Total Environ 763:143007 ArticleGoogle Scholar
- Kabeyi MJB, Olanrewaju OA (2022) Biogas production and applications in the sustainable energy transition. J Energy 2022:1–43 ArticleGoogle Scholar
- Kovačić Đ, Lončarić Z, Jović J, Samac D, Popović B, Tišma M (2022) Digestate management and processing practices: a review. Appl Sci 12(18):9216 ArticleGoogle Scholar
- Tse TJ, Wiens DJ, Chicilo F, Purdy SK, Reaney MJT (2021) Value-added products from ethanol fermentation—a review. Fermentation 7(4):267 ArticleGoogle Scholar
- Pati S, De S, Chowdhury R (2023) Integrated techno-economic, investment risk and life cycle analysis of Indian lignocellulosic biomass valorisation via co-gasification and syngas fermentation. J Clean Prod 423:138744 ArticleGoogle Scholar
- Manhongo TT, Chimphango A, Thornley P, Röder M (2021) Techno-economic and environmental evaluation of integrated mango waste biorefineries. J Clean Prod 325:129335 ArticleGoogle Scholar
- Catalán E, Sánchez A (2020) Solid-state fermentation (SSF) versus submerged fermentation (SmF) for the recovery of cellulases from coffee husks: a life cycle assessment (LCA) based comparison. Energies 13(11):2685 ArticleGoogle Scholar
- Demichelis F, Tommasi T, Deorsola FA, Marchisio D, Mancini G, Fino D (2022) Life cycle assessment and life cycle costing of advanced anaerobic digestion of organic fraction municipal solid waste. Chemosphere 289:133058 ArticleGoogle Scholar
- Ubando AT, Rivera DRT, Chen W-H, Culaba AB (2020) Life cycle assessment of torrefied microalgal biomass using torrefaction severity index with the consideration of up-scaling production. Renew Energy 162:1113–1124 ArticleGoogle Scholar
- Thengane SK, Burek J, Kung KS, Ghoniem AF, Sanchez DL (2020) Life cycle assessment of rice husk torrefaction and prospects for decentralized facilities at rice mills. J Clean Prod 275:123177 ArticleGoogle Scholar
- Makepa DC, Chihobo CH, Manhongo TT, Musademba D (2023) Life-cycle assessment of microwave-assisted pyrolysis of pine sawdust as an emerging technology for biodiesel production. Results Eng 20:101480 ArticleGoogle Scholar
- Yang X, Han D, Zhao Y, Li R, Wu Y (2020) Environmental evaluation of a distributed-centralized biomass pyrolysis system: a case study in Shandong, China. Sci Total Environ 716:136915 ArticleGoogle Scholar
- Wang Y, Yang Y (2022) Research on greenhouse gas emissions and economic assessment of biomass gasification power generation technology in China based on LCA method. Sustainability 14(24):16729 ArticleGoogle Scholar
- Ochieng R, Gebremedhin A, Sarker S (2022) Integration of waste to bioenergy conversion systems: a critical review. Energies 15(7):2697 ArticleGoogle Scholar
- Velvizhi G, Balakumar K, Shetti NP, Ahmad E, Pant KK, Aminabhavi TM (2022) Integrated biorefinery processes for conversion of lignocellulosic biomass to value added materials: paving a path towards circular economy. Bioresour Technol 343:126151 ArticleGoogle Scholar
- Doddapaneni TRKC, Ahmad F, Valgepea K, Kikas T (2022) Integrated thermochemical and biochemical processes for the production of biofuels and biochemicals. Biomass Biofuels Biochem:67–105
- Madejski P, Chmiel K, Subramanian N, Kuś T (2022) Methods and techniques for CO2 capture: review of potential solutions and applications in modern energy technologies. Energies 15(3):887 ArticleGoogle Scholar
- Yang B, Shao C, Hu X, Ngata MR, Aminu MD (2023) Advances in carbon dioxide storage projects: assessment and perspectives. Energy Fuel 37(3):1757–1776 ArticleGoogle Scholar
- Jenkins C (2020) The state of the art in monitoring and verification: an update five years on. Int J Greenh Gas Control 100:103118 ArticleGoogle Scholar
- Sun S, Sun H, Williams PT, Wu C (2021) Recent advances in integrated CO 2 capture and utilization: a review. Sustainable Energy Fuels 5(18):4546–4559 ArticleGoogle Scholar
- Podder J, Patra BR, Pattnaik F, Nanda S, Dalai AK (2023) A review of carbon capture and valorization technologies. Energies 16(6):2589 ArticleGoogle Scholar
- Akinola TE, Prado PLB, Wang M (2022) Experimental studies, molecular simulation and process modelling\simulation of adsorption-based post-combustion carbon capture for power plants: a state-of-the-art review. Appl Energy 317:119156 ArticleGoogle Scholar
- Yadav S, Mondal SS (2022) A review on the progress and prospects of oxy-fuel carbon capture and sequestration (CCS) technology. Fuel 308:122057 ArticleGoogle Scholar
- Gao W et al (2020) Industrial carbon dioxide capture and utilization: state of the art and future challenges. Chem Soc Rev 49(23):8584–8686 ArticleGoogle Scholar
- Simonsen KR, Hansen DS, Pedersen S (2024) Challenges in CO2 transportation: trends and perspectives. Renew Sust Energ Rev 191:114149 ArticleGoogle Scholar
- Liu E, Lu X, Wang D (2023) A systematic review of carbon capture, utilization and storage: status, progress and challenges. Energies 16(6):2865 ArticleGoogle Scholar
- Al Baroudi H, Awoyomi A, Patchigolla K, Jonnalagadda K, Anthony EJ (2021) A review of large-scale CO2 shipping and marine emissions management for carbon capture, utilisation and storage. Appl Energy 287:116510 ArticleGoogle Scholar
- Cao C, Liu H, Hou Z, Mehmood F, Liao J, Feng W (2020) A review of CO2 storage in view of safety and cost-effectiveness. Energies 13(3):600 ArticleGoogle Scholar
- Haq B, Muhammed NS, Liu J, Chua HT (2023) Enhanced natural gas production using CO2 injection: application to sustainable hydrogen production. Fuel 347:128474 ArticleGoogle Scholar
- Rycroft L et al (2024) Geological storage of CO2. In: Deployment of Carbon Capture and Storage. Elsevier, pp 133–266 ChapterGoogle Scholar
- Mwakipunda GC et al (2023) Recent advances in carbon dioxide sequestration in deep unmineable coal seams using CO2-ECBM technology: experimental studies, simulation, and field applications. Energy Fuel 37(22):17161–17186 ArticleGoogle Scholar
- Paltsev S, Morris J, Kheshgi H, Herzog H (2021) Hard-to-abate sectors: the role of industrial carbon capture and storage (CCS) in emission mitigation. Appl Energy 300:117322 ArticleGoogle Scholar
- Mahjour SK, Faroughi SA (2023) Risks and uncertainties in carbon capture, transport, and storage projects: a comprehensive review. Gas Sci Eng:205117
- Lau HC, Ramakrishna S, Zhang K, Radhamani AV (2021) The role of carbon capture and storage in the energy transition. Energy Fuel 35(9):7364–7386 ArticleGoogle Scholar
- Zapantis A, Townsend A, Rassool D (2019) Policy priorities to incentivise large scale deployment of CCS. In: Thought Leadership Report. Global CCS Institute Google Scholar
- Romanak K, Dixon T (2022) CO2 storage guidelines and the science of monitoring: achieving project success under the California Low Carbon Fuel Standard CCS Protocol and other global regulations. Int J Greenh Gas Control 113:103523 ArticleGoogle Scholar
- Sun X et al (2021) Hubs and clusters approach to unlock the development of carbon capture and storage–case study in Spain. Appl Energy 300:117418 ArticleGoogle Scholar
- Wei N, Li X, Liu S, Lu S, Jiao Z (2021) A strategic framework for commercialization of carbon capture, geological utilization, and storage technology in China. Int J Greenh Gas Control 110:103420 ArticleGoogle Scholar
- Babin A, Vaneeckhaute C, Iliuta MC (2021) Potential and challenges of bioenergy with carbon capture and storage as a carbon-negative energy source: a review. Biomass Bioenergy 146:105968 ArticleGoogle Scholar
- Å. Slagtern et al., “Recent activities of the Technical Group of the Carbon Sequestration Leadership Forum (CSLF),” in 14th Greenhouse Gas Control Technologies Conference Melbourne, 2018, pp. 21–26.
- Williams RH (1998) Fuel decarbonization for fuel cell applications and sequestration of the separated CO2. Ecorestruct Implicat Sustain Dev
- Pires JCM (2019) Negative emissions technologies: a complementary solution for climate change mitigation. Sci Total Environ 672:502–514 ArticleGoogle Scholar
- Almena A, Thornley P, Chong K, Röder M (2022) Carbon dioxide removal potential from decentralised bioenergy with carbon capture and storage (BECCS) and the relevance of operational choices. Biomass Bioenergy 159:106406. https://doi.org/10.1016/j.biombioe.2022.106406ArticleGoogle Scholar
- Palmer J, Carton W (2021) Carbon removal as carbon revival? Bioenergy, negative emissions, and the politics of alternative energy futures. Front Clim 3:678031 ArticleGoogle Scholar
- Iyer J et al (2022) A review of well integrity based on field experience at carbon utilization and storage sites. Int J Greenh Gas Control 113:103533 ArticleGoogle Scholar
- Zahraee SM, Shiwakoti N, Stasinopoulos P (2020) Biomass supply chain environmental and socio-economic analysis: 40-years comprehensive review of methods, decision issues, sustainability challenges, and the way forward. Biomass Bioenergy 142:105777 ArticleGoogle Scholar
- Yan J et al (2020) Characterizing variability in lignocellulosic biomass: a review. ACS Sustain Chem Eng 8(22):8059–8085 ArticleGoogle Scholar
- McLaughlin H et al (2023) Carbon capture utilization and storage in review: sociotechnical implications for a carbon reliant world. Renew Sust Energ Rev 177:113215 ArticleGoogle Scholar
- Emenike O, Michailos S, Finney KN, Hughes KJ, Ingham D, Pourkashanian M (2020) Initial techno-economic screening of BECCS technologies in power generation for a range of biomass feedstock. Sustain Energy Technol Assess 40:100743 Google Scholar
- Smith P et al (2016) Biophysical and economic limits to negative CO2 emissions. Nat Clim Chang 6(1):42–50 ArticleGoogle Scholar
- Kearns D, Liu H, Consoli C (2021) Technology readiness and costs of CCS, vol 3. Global CCS institute Google Scholar
- Hepburn C et al (2019) The technological and economic prospects for CO2 utilization and removal. Nature 575(7781):87–97 ArticleGoogle Scholar
- Fuss S et al (2014) Betting on negative emissions. Nat Clim Chang 4(10):850–853 ArticleGoogle Scholar
- Volkart K, Bauer C, Boulet C (2013) Life cycle assessment of carbon capture and storage in power generation and industry in Europe. Int J Greenh Gas Control 16:91–106 ArticleGoogle Scholar
- Yang B, Wei Y-M, Hou Y, Li H, Wang P (2019) Life cycle environmental impact assessment of fuel mix-based biomass co-firing plants with CO2 capture and storage. Appl Energy 252:113483. https://doi.org/10.1016/j.apenergy.2019.113483ArticleGoogle Scholar
- He Y, Zhu L, Fan J, Li L, Liu G (2021) Life cycle assessment of CO2 emission reduction potential of carbon capture and utilization for liquid fuel and power cogeneration. Fuel Process Technol 221:106924. https://doi.org/10.1016/j.fuproc.2021.106924ArticleGoogle Scholar
- Varling AS, Christensen TH, Bisinella V (2023) Life cycle assessment of alternative biogas utilisations, including carbon capture and storage or utilisation. Waste Manag 157:168–179. https://doi.org/10.1016/j.wasman.2022.12.005ArticleGoogle Scholar
- Bennett JA, Melara AJ, Colosi LM, Clarens AF (2019) Life cycle analysis of power cycle configurations in bioenergy with carbon capture and storage. Procedia CIRP 80:340–345 ArticleGoogle Scholar
- ApSimon H, Oxley T, Woodward H, Mehlig D, Holland M, Reeves S (2023) Integrated assessment modelling of future air quality in the UK to 2050 and synergies with net-zero strategies. Atmosphere 14(3):525 ArticleGoogle Scholar
- Al-Qayim K, Nimmo W, Pourkashanian M (2015) Comparative techno-economic assessment of biomass and coal with CCS technologies in a pulverized combustion power plant in the United Kingdom. Int J Greenh Gas Control 43:82–92 ArticleGoogle Scholar
- Weihs GAF et al (2022) Life cycle assessment of co-firing coal and wood waste for bio-energy with carbon capture and storage–New South Wales study. Energy Convers Manag 273:116406 ArticleGoogle Scholar
Acknowledgements
The authors would like to express their sincere gratitude to the Department of Fuels and Energy and Chinhoyi University of Technology for rendering all the indispensable support needed in this work.